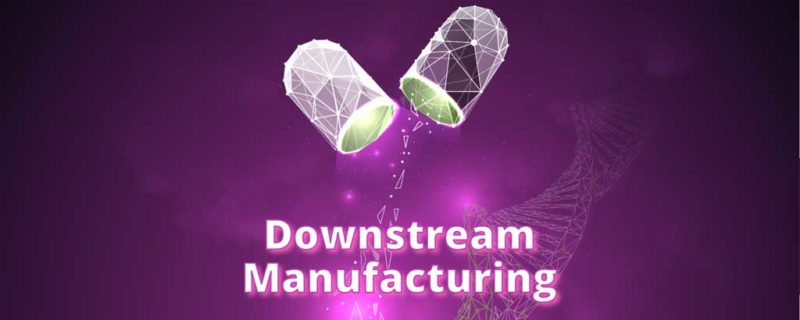
Downstream Manufacturing of Gene Therapy Vectors
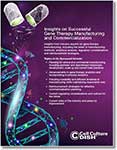
“Insights on Successful Gene Therapy Manufacturing and Commercialization”.
You can download all the articles in the series, by downloading the eBook.
Introduction
The downstream manufacturing process takes the output from upstream operations and transforms it through multiple steps into a viable drug product ready for administration to patients. The major challenge in downstream processing is to maximize yield while meeting both product and impurity specifications. Viral vectors destined for clinical use must comply with regulatory standards for product safety and potency and this means contaminants must be removed and impurities controlled.
Viral Vectors
Viral vectors vary greatly in physical characteristics depending on the type of virus. Approaches to vector purification exploit the physical characteristics of the viral particle such as size, surface charge and hydrophobicity. The wide variation in physical characteristics between various vectors presents a diverse set of purification challenges. This diversity results in a multiplicity of needed techniques for production and purification, which also presents a challenge in development and operation of multi-use facilities, such as those routinely operated by contract manufacturing organizations. Table 1 shows some similarities and differences for commonly used virus vectors: adenovirus (AdV), adeno-associated virus (AAV) and retroviruses (which are primarily gammaretrovirus) (RV), and lentivirus (a type of retrovirus) (LV).
Table 1. Differences and similarities in four common viruses used as viral vectors
Virus | Size (nm) | Envelope | Stability | Buoyant Density |
---|---|---|---|---|
Adenovirus | ~90 | No | High | 1.34 (CsCl) |
AAV | ~ 20 | No | High | 1.41 (CsCl) |
Retrovirus/Lentivirus | ~90-120 | Yes | Low(a) | 1.16 (Sucrose) |
Further challenges arise in that there is variation in physical characteristics not only between the different vectors, but also between vector serotypes. These differences can be small, as in between AAV serotypes, however they can have major implications to the downstream process conditions. Moreover, physical differences can vary within the same vector due to variation in the transgene insert.
Process differences also present further challenges. For example, AAV accumulates both in the media and intracellularly via a partially lytic process. The amount of cell lysis is dependent on the AAV subtype. In order to improve yield for some AAV subtypes, cells are lysed via detergent. The cell lysis, in turn, can significantly increase the quantities of host cell contaminants including DNA and protein that increase purification burden.
Another issue in AAV purification is the removal of “empty” capsids devoid of transgene. The high proportion of empty capsids, which can be as high as 95%, makes this even more difficult.2 Currently, the role of empty capsids for a therapeutic is unclear: there are reports that show that empty capsids in an AAV therapeutic can either be clinically beneficial or detrimental depending on the circumstance.3-5 Despite the ongoing debate over a possible benefit from the presence of empty capsids in AAV therapies, they are still considered a process related impurity, and as such the level of empty capsids must be controlled. Additionally, partially filled AAV capsids are a potential safety concern.
RV/LV vectors present their own unique purification challenges. The presence of a lipid envelope makes these vectors less stable and more shear sensitive. The instability of these particles can lead to a loss of yield – potentially up to 70%.6 It is not uncommon for LV to have a half-life of just 8-12 hours.7 So designing the downstream process to minimize time, for instance reducing intermediate hold time, is a critical consideration.
Difficulties in the purification of vectors can arise because of vector type or choice of upstream platform and process. For example: 1) vector supernatants that are produced by transfection contain large amounts of contaminating plasmid DNA or 2) use of a suspension cell bioreactor can produce more debris than a fixed-bed bioreactor or 3) intracellular viruses produce more debris than excreted viruses or 4) large viruses, such as herpes, may not be filter sterilizable, and may require aseptic processing, and the validation that goes with that.8-10 The use of serum-supplemented cell culture media can also cause a greater purification burden and increased viscosity from sera can affect downstream process efficiency.11
In this regard, upstream production systems using serum-free media, which is common for stirred tank bioreactors, can ease the downstream purification burden. Another consideration is that fixed bed bioreactors generally produce output with reduced turbidities. This can also reduce the downstream burden, particularly upon clarification.
Despite the differences in physical characteristics between vectors, current downstream protocols generally have a similar workflow: A clarification step (which may require additional depth filtration due to cell lysis at time of harvest), purification (typically ion exchange or affinity chromatography), and a polishing step (additional chromatography step/size exclusion) (Figure 1). In addition to the above, filtration, dialysis, or TFF steps are typically utilized in order to concentrate, exchange buffer, and prepare final formulation.
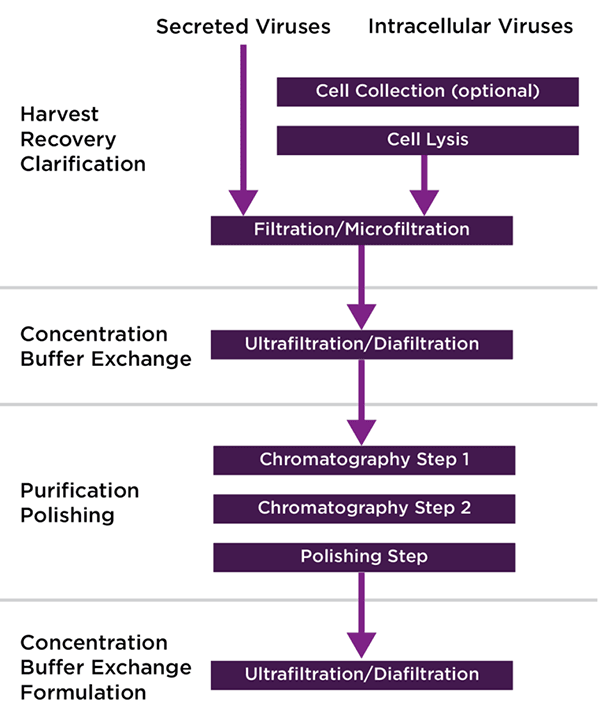
The choice of viral vector will have an impact of the purification steps that are required. For example, AAV purification typically involves an affinity chromatography step to remove contaminants and an ion exchange chromatography (IEC) step for the separation of empty and full capsids. LV purification, on the other hand, employs mostly one, sometimes two chromatography steps.
Cell lysis, clarification, and filtration
Cell lysis is often required to improve viral yield when the vector is not fully secreted by the cells (e.g. AdV, AAV). Lysis can be performed by physical disruption via microfluidization or heat-shock treatments. Alternatively, detergents such Triton™ X-100 have proven efficient for lysis in production of AdV and AAV and has been utilized for insect cell vectors as well.12 However, the European REACH list of banned substances limits the use of alkylphenol ethoxylate (APE) surfactants such as nonylphenol ethoxylates (NPEs) and octylphenol ethoxylates (OPEs), which include Triton X-100 and these are scheduled for severe restriction in 2021.13
Alternative detergent lysis approaches can usually be developed using Tween™ detergents14 and there are analytical methods for the detection of Tween 20 in vector preparations thereby providing a method to show that the detergent has been removed during downstream processing. Any cell lysis method requires optimization as lysis is highly dependent on the cell concentration, incubation time, incubation temperature, cell type, and the virus being released.14
Clarification involves the initial removal of cell debris and impurities and typically involves a combination of depth filters and sterile filtration. This approach can also contribute to the overall bioburden control strategy. To improve recovery, direct flow solutions for clarification have been utilized with some vectors using 1 µm > 0.8 µm > 0.45 µm filters.15 Note that the sterile filtration of some viruses may be challenging particularly for large enveloped viruses. Another key consideration, when the downstream harvest stream has high turbidity, is the inclusion of a pre-filter with relatively large nominal pore sizes to remove larger cellular debris and protect subsequent filters from clogging and creating high pressure in the subsequent purification step.
Alternatively, tangential flow filtration (TFF) has proven an effective method for both clarification and concentration with reports of recoveries as high as 90–100%, although flow rate may need to be adjusted.16,17 Reduction in viral yield during concentration and diafiltration, due to loss of stability or loss of function, is of upmost concern, particularly for enveloped viruses such as RV/LV vectors. Strategies may need to be developed to minimize shear throughout the purification processes, such as limiting flow rates or employing single-pass TFF, which is an area of active development that has the potential to improve yields. The duration of the process is always a concern for unstable viruses
The limitation of classical centrifuge methods
Purification of small quantities of virus for research in academia or for small clinical studies has classically relied heavily on ultracentrifugation methods. Historically CsCl has been commonly used for AAV and AdV ultracentrifugation. However, iodixanol is now preferred because it is easier to dialyze out, leads to less aggregation, and run times are shortened from 24 h to just a few hours.18 Because of the decreased stability of enveloped viruses such as RV/LV, sucrose is generally used for density purification of these viruses.
A limitation of density ultracentrifugation is scale up due to volume limitations, operational logistics and cost. Since larger volume ultracentrifuges are now commercially available, it is possible to at least consider ultracentrifugation at some scales. However, any benefit must be weighed against the disadvantages of labor, processing time, and capital expenditure along with health and safety issues associated with large scale ultracentrifugation of infectious virus.11 Multiple rounds of gradient ultracentrifugation may be required to highly purify particles.
Ultracentrifugation has been used by a number of companies and academic institutions to produce clinical material at a small scale and ultracentrifugation remains in use in current processes to some degree to separate product related impurities such as empty capsids and non-product impurities such as HCP. Ultracentrifugation may have some application in the later stages of an overall purification scheme.11
Chromatography
Chromatographic separation is a more versatile and powerful method for large scale production of recombinant adenovirus or AAV compared to classical centrifugation19. In addition, chromatography can be an effective means of removing potential adventitious viruses as they can have different physical properties than the vector on many chromatographic materials. Chromatography is widely used and may be applied in various downstream steps, including capture, concentration, purification, and polishing steps.20
Approaches that have been developed for monoclonal antibodies (mAb) and recombinant proteins have been modified for the purification of viruses. However, resins that are the backbone of mAb processing are inherently disadvantaged by the diffusion limits of the pores in the media. The pores are often so small that they exclude viruses, limiting the binding capacity and typically require longer residence times. This, in turn, means operating at lower flow rates and increasing processing times. Moreover, resins typically require packing and validation, which is laborious. This weakness has led to an increased adoption of chromatographic approaches that are not limited by diffusion and that can be operated convectively, this includes monoliths and membranes.
Both monoliths and membranes are available in pre-packed formats and may have “plug and play” advantages. Typically process economics are more challenging for monoliths and this has furthered the adoption of membrane chromatography. Membrane adsorbers integrate easily with other unit operations and deliver highly productive downstream operations. Membrane adsorbers are increasingly used in new flexible manufacturing facilities, in which speed of operations and robust performance are essential production criteria. They can result in a more productive downstream operation which, in some applications, can be an order of magnitude greater than resins.21 Furthermore, the membranes can come pre-packed, such as the Mustang® Q Membrane (Pall Corporation), and have demonstrated scalability due to their multilayer membrane approach, which is held at a constant thickness per bed depth.22 Moreover, the larger pore size of membranes at ~0.8 µm facilitates the purification of viruses and removal of DNA.22
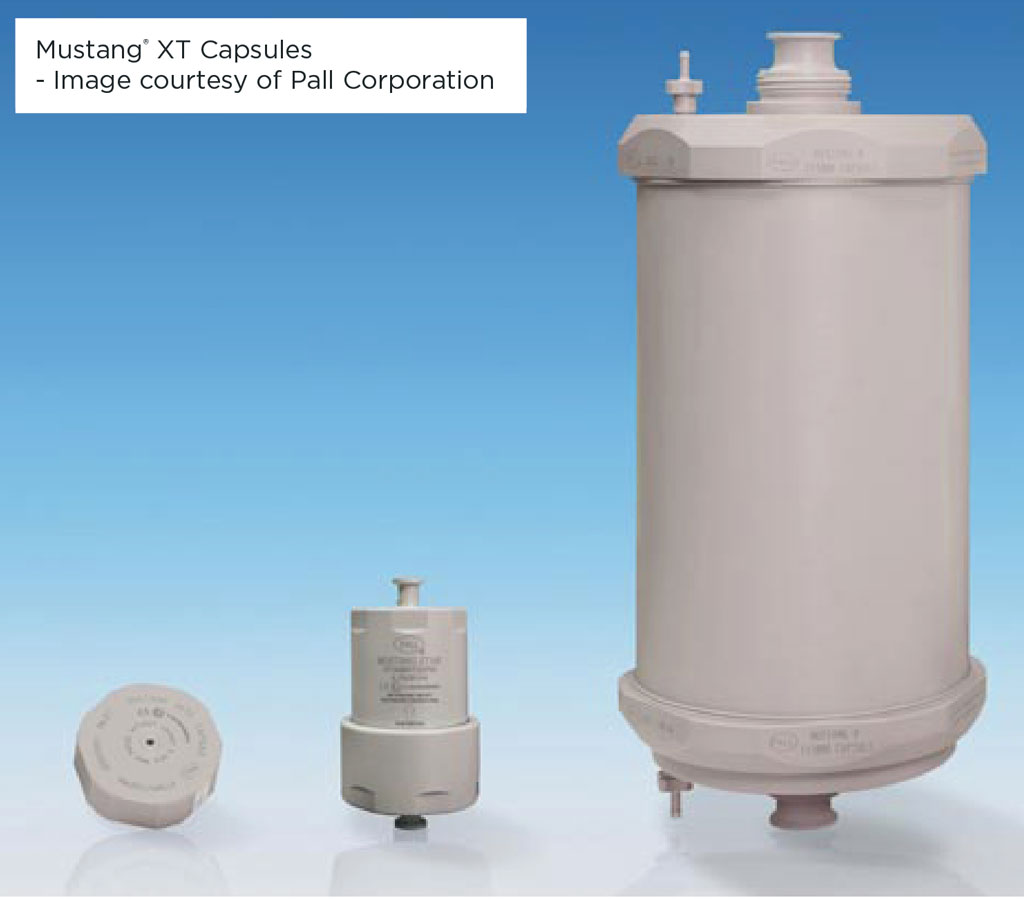
Affinity chromatography
Affinity chromatography (AC) relies on the interaction of the viral particle with a ligand that has a high affinity for the target, but not for contaminants.
For viral vectors two affinity approaches are currently used. Heparin and affinity approaches are used for AAV purification, which primarily rely upon camelid-derived ligands. Heparin affinity chromatography has also been used for purification of LV and other viruses at scale.23 The use of low ionic strength solutions can help preserve vector integrity.24 However, there can be impurities that bind non-specifically, the removal of which necessitates an additional purification step.23 Other potential drawbacks are animal derived ligands and the preferred selectivity of some AAV serotypes.25 Cellufine™ sulphated cellulose (AMSBIO) is an alternative to heparin with similar specificity for purification of various viruses.26
For AAV, AVB Sepharose™ affinity resin (Cytiva) utilizes a recombinant protein ligand and has been shown to be an effective strategy for the purification of AAV serotypes 1, 2, 3, and 5 as recommended by the manufacturer.27 Others have reported its successful use with other serotypes, although efficiency may vary.28,29 AVB resin has been successfully used in combination with IEC to purify a variety of AAV serotypes. Furthermore, POROS™ CaptureSelect™ AAVX affinity resin (Thermofisher) has been developed for a broad range of serotypes (AAVX for 1-8, AAVrh 10, and some synthetic serotypes),. Additionally there are Poros resins developed for AAV8 and AAV9 specifically.
These affinity matrices reportedly also lead to an increase in the overall yield3, and a lower cost of goods.30 Genethon has reported purification of AAV9 using CaptureSelect AAV9 resin at 10L and 50L scale with >80% recovery.19
These affinity resins provide a major step forward in ease of development for AAV purification, however there are a series of limitations that remain. These include: 1) possible leakage of ligand into the vector preparation that may require additional analytical testing or additional purification steps; 2) lack of specificity between empty and full capsids, which necessitates additional steps such as ion-exchange chromatography for that purpose.28; and 3) the protein-based affinity ligand is not amenable to cleaning via NaOH. This limits column regeneration through many cycles and makes cycling of the resin a challenge.7 Extensive studies must be conducted just to obtain a few cycles of regeneration. Thus, this lack of regeneration of current AAV protein-based affinity resins adds cost to their use.
Ion exchange chromatography
Ion exchange chromatography (IEC) is a simple, versatile, and cost-effective technique that has emerged as a critical step in the purification of many vectors. Anion (AEX) or cation (CEX) exchangers can be used to bind either positively or negatively charged viruses, respectively. IEC can be used in both bind/elute mode (example: virus capture or HCP removal) or flow through mode (example: removal of DNA) and it has found an important role for both DNA and HCP removal. IEC has also found an important role in virus reduction in the monoclonal industry. The features of high capacity, high resolution, and high salt tolerance make IEC especially suited to bioprocessing applications.
AEX chromatography is applied to both AAV and LV processing. Typically, LV purification relies on a single AEX chromatography step. Here there is a big advantage of membrane chromatography as lentivirus is so large that is cannot access resin pores. This results in lower capacities for resins.
AEX can purify LV vectors of high purity directly from the crude clarified harvest, although the use of high ionic strength solutions during the elution step can reduce LV yield.31 IEC can also be used to separate helper viruses such as AdV and baculovirus from AAV preparations.32 IEC has also been applied to the capture step for large scale production of AAV. This method has been used for the generation of GMP AAV vector.3,33,34
AEX is one of the few methods that can separate empty from full AAV and AdV capsids because of a slight physical difference from the presence of full-length DNA.35 Some reports show >90% elimination of empty capsids.35-37
To date, AEX has been demonstrated to be effective across many AAV serotypes and it could potentially allow the separation of empty capsids of all AAV serotypes.28 AEX has also proven to be an efficient method at larger scale.3 However, the major challenge for the use of AEX for the separation of empty AAV capsids is that operating conditions need to be optimized for each individual serotype, and sometimes even between different vectors of the same serotype. Substantial development time (up to many months) may be required to optimize the gradients required to achieve the separation. Anything that affects the charge of a vector, such as serotype, vector design, or length of the transgene insert, typically requires re-optimization of the gradient conditions. Thus, at the present time, one of the major challenges in gene therapy development is the effort required to optimize the AAV full capsid enrichment. Furthermore, there are often technical challenges for IEC to fully remove empty capsids from preparations with a high empty capsid load (i.e. 90%).
Recent advancements in empty/full AAV separation include the use of Mustang Q membrane to separate empty from full capsids. In their patent pending approach, Pall Biotech found that a step salt gradient provided greater empty/full separation than a linear gradient. An initial ~ 1 mS step on a small format XT Acrodisc was used to initially probe separation of empty/full capsids and examine the profile of the process as shown by the differences in 280 nm (protein) and 260 nm (nucleic acid) adsorption profile. Absorbance is one of a handful of methods commonly used as an assay for the relative amounts of empty/full AAV capsids.38 The process was then scaled to the 5 ml capsule with identical results (Figure 2). Furthermore, Pall noted that the gradient could be reduced to 2-steps so that the process would be easier to implement in a manufacturing environment. Thus, work with Mustang Q AEX shows that it is possible to implement separation of AAV empty/full capsids using formats that can have distinct advantages over resins.
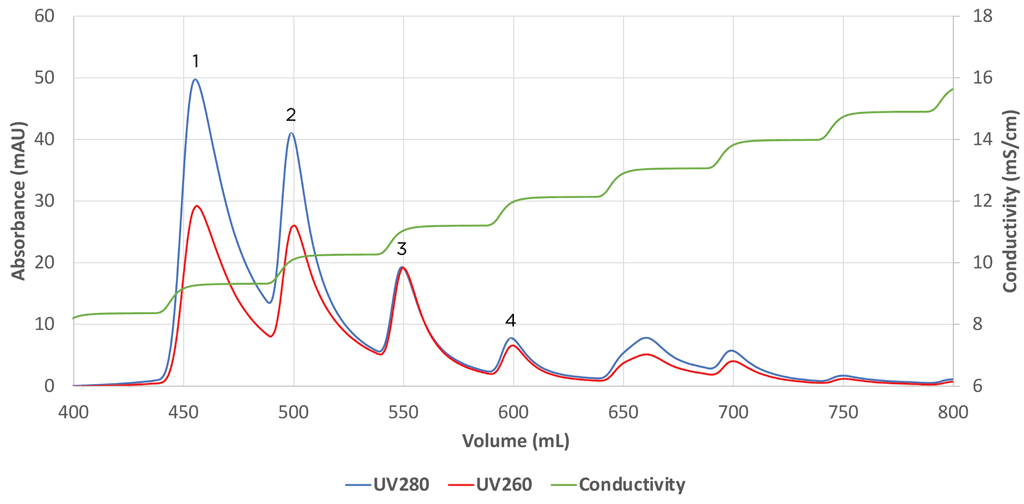
Separation of empty/full AAV5 capsids using Mustang Q XT5. Initial elution peaks shown by Absorbance at 280nm and 260nm contain primarily empty AAV5 capsids while peaks with similar absorbance intensities (peaks 3 and 4) contain primarily full AAV5 capsids.
Size exclusion chromatography
Size exclusion chromatography (SEC) can be used to separate virus particles from contaminants on the basis of size and shape.39 SEC has benefits in that it is very gentle on particles since there is no binding or elution and it is performed isocratically. However, SEC may not be suitable for large-scale processing as it is not usually possible for the load to be larger than 10% of the resin volume. Additionally, the SEC resins used in bioprocessing are compressible, this limits column size and flow rate. Nevertheless, SEC has found use as a final polishing for many viruses including AAV and LV.9,39,40
Hydrophobic interaction chromatography
Hydrophobic interaction chromatography (HIC) been used for viral capture/clearance in the recombinant protein industry for years. In purification schemes, HIC has been used for the purification both AdV and AAV2.41,42 However, its use with different serotypes of AAV has not been extensively documented. HIC has challenges. The use of high ionic strength solutions required during binding can affect the stability of some viruses41 and another step must be developed to remove the high salt concentration. Generally, HIC is not employed much in current day vector downstream processes.
Concentration/buffer exchange
Concentrating viruses by ultracentrifugation can provide effective concentrations and high yields for some viruses. However, ultracentrifugation has potential disadvantages such as losses of functional vector particles due to shear stress43 and co-concentration of impurities.40 The use of low-speed centrifugation for longer durations is a more gentle approach, which may prove valuable with less stable vectors for higher infectious particle recoveries.44 As discussed above, centrifugation methods have impractical scalability and alternative methods are typically used for industrial production.
Tangential Flow Filtration (TFF) has advantages for concentration or buffer exchange in that it is typically scalable and allows for mild processing conditions for use with less stable vectors. Products are available in numerous configurations based on the molecular weight cutoff, the nature of the membranes, or the filter surface. Although the achievable concentration factors are often lower than for centrifugation methods there is an additional benefit in that some impurities may be removed during the process of concentration.16,45
Tangential Flow Filtration (TFF)
TFF technology can be used in several places throughout the downstream process. The separation is mainly based on size, although other molecular properties such as surface charge and hydrophobicity play some role as well. In some applications (e.g. continuous harvest) filters are used with pore sizes that allow passage of the product and retain larger impurities (e.g. intact cells, cellular debris). In other applications (e.g. concentrating pools upstream of chromatography to limit load times, or processing purified pools into final formulation) the product is retained on the upstream side of the filter and smaller impurities and buffer components are filtered out. Tangential flow filtration is a technology that has transferred well from the recombinant protein industry. Compared to alternative techniques, such as centrifugation, it has shown good scalability, process economics, process robustness, and in many cases can provide yields near or above 90%. The decades of prior knowledge also aid in efficient process development, process characterization, scale-up, and tech transfer.
Generally speaking, TFF filters are commercially available in two main formats: flat-sheet cassettes and hollow fiber modules. Both formats are widely used in gene therapy applications. An advantage of flat-sheet cassettes is that they tend to provide a higher flux than hollow fiber modules. Flat-sheet TFF also offers a ‘modular’ approach in that more filtration area can be added by simply ‘stacking’ more cassettes together in the filter holder. Hollow fiber TFF is thought to exhibit lower shear than flat-sheet cassettes, which is an important consideration for the downstream processing of fragile biomolecules. Lentivirus is especially susceptible to shear damage.
Most TFF membranes are caustic stable and can be run in closed, aseptic processes – a key consideration for gene therapy manufacturing. There are several newer TFF products on the market, in both flat sheet and hollow fiber format, that are provided with a sterile claim, and in some cases can be connected into the system with aseptic connectors. This can provide the same closed processing benefits along with less required flushing and therefore reduced operator time and footprint.
Finally, as gene therapy products are often more sensitive to environmental conditions such as shear and time compared to mAbs and many recombinant proteins, the industry will likely continue to investigate single-pass TFF technology. There are products on the market for single-pass concentration and diafiltration such as the Cadence™ Inline Concentrator and Inline Diafiltration Module (Pall Corporation). These technologies can offer reduced shear by limiting pump passes, and reduced processing time by combining unit operations continuously.
Bioburden Control
Bioburden control throughout the manufacturing processes is a critical part of GMP compliance and minimizes the risk of microbial contamination of the final product. In most processes the final vector preparation is filtered by one, or more 0.2 µm sterilizing grade filters as a component of control, although there may be variations in its placement in downstream protocols.3 Some vector products are not filter sterilizable at 0.2 µm because of the size of the virus. It is possible to skip sterile filtration provided that the process can be certified as being fully aseptic; however, this requires operation of a closed process and operations must be performed in a clean room.8 TFF systems can provide increased throughput for sterilization and can have benefit in that regard. Nevertheless, sterile filtration currently can be a huge challenge for some unstable viruses as they can lose function.
Insights on downstream process issues
Moving a downstream process to clinical and commercial manufacturing
Transfer of a vector from a developmental setting in academia, to manufacturing for clinical use, presents several considerations for downstream production development. Often the objectives and consequently choice of tools and technologies are different between academia and clinical production. Areas including consistency of operation and bioburden control are not typically focused on in most research production but are critical in clinical manufacturing. Thus, there are several areas that need further examination and possible alternative solutions.
Scalability
One of the major considerations when moving from research production is how to achieve the increased production scale. At larger scales, purification methods need to accommodate large volumes with high efficiency and speed. One approach toward implementation of greater scale is to move away from poorly scalable centrifugation-based techniques to systems with greater throughput, such as filtration. Another approach is the use of chromatographic approaches as they are considered to be one of the best technological methods for manufacturing applications. The scalability of IEC or AC methods can provide effective purification of many vectors.11,35 When considering individual chromatographic approaches, efficiency is a huge aspect as it can lead to a smaller, simpler process and greater scale. It has been shown that VSG-g LV vectors can be purified using Mustang Q membrane (Pall Corporation) up to a volume of 1,500 L/day46 and lead to vector preparations of high purity; in contrast, LV recovery may not be as efficient as with ultracentrifugation due to the fragility of these vectors.
GMP Compliance
Research material is not required to be GMP compliant, thus this is a major change when moving a product to clinical manufacturing. Vectors for therapeutic use must meet stringent standards for purity and safety with product quality of upmost importance. This transition to GMP requires examination of the existing process including raw materials and reagents. Rational design of the downstream process to simplify and increase efficiency can reduce the number of required GMP reagents and steps, which increases quality, safety and significantly lowers costs.
Adventitious agent control is an aspect of GMP manufacturing that is focused on once a process has moved into clinical production. Adventitious virus control can be particularly challenging since the product is also a virus. Use of sterile filtration can cause concern as many viruses are unstable or are difficult to filter because of viral size. Loss of yield during filtration continues to be a significant challenge for many vectors, including LV. Development of alternative methods for agent control, such as chromatographic approaches, would benefit the industry.
Process development timelines
The timeline to develop a downstream process is dependent on many factors such as the type of vector, intended use, scale, cost considerations, and technology available. Independent of the factors involved, a reduced developmental timeline is a desirable goal as it quickens time to market and lowers cost. Note that time required for scientific work on a downstream process can be extensive, several months, depending on the resources available, as there are few “platform-like” or “plug and play” solutions currently on the market. There have been helpful advances, however, such as the rise of vector-specific AC solutions.19 For AAV developing the process of empty/full capsid separation takes a long time. It can also be expensive as it can consume considerable feedstock at scale.
Costs considerations
Costs are a combination of many factors such as, time, labor, operating expenses, and capital expenses. The technology used in downstream processing can impact the timeline of process development, product quality, ease of process scale-up, and cost of goods (COGs).19 Replacing traditional, academic methods that are time consuming, complex, and inefficient with approaches that are more cost effective, such as chromatography, can lower costs with the tradeoff of required redevelopment time.20
Implementing newer strategies such as IEC or AC that have greater potential for throughput and scale up can significantly simplify process strategy and increase efficiency. These purification schemes significantly shorten process development time and reduce COGs. In that regard, the newer affinity chromatography approaches, if applicable to the particular vector, can potentially simplify the process architecture to a single chromatography capture step accompanied only by a clarification step and a polishing step (AAV requires an additional empty/full separation step). The use of purification and polishing chromatographic systems can result in high purity with many vectors.11
Development and use of technologies such as (semi)-continuous processes can result in footprint reduction, increased productivity, automation, reduced inventory and storage, and fewer unit operations – all of which can lower costs.20,47 Other cost cutting strategies, include reduction of the chromatographic unit operations, through the use of multi-column systems or single-use systems20 and chromatographic column cycling with expensive resins. Another consideration is implementing scalable solutions for downstream, such as depth filtration, chromatography, etc.
The use of tangential flow filtration (TFF), which can be up-scaled in manufacturing, can provide cost savings. In that regard, automated TFF systems are available to control both the transmembrane pressure as well as the level of concentration. Additionally, automation of TFF can provide an ergonomic and secure environment for the operator and facilitate the speed and scale up of the process. Often a 20–80x concentration factor can easily be achieved using these systems.11
Risk Assessment
Another important consideration is an overall evaluation of vector risks (risk assessment) and subsequent design of the upstream and downstream process to minimize that risk with available technology. For example, genotoxicity and immunotoxicity are two theoretical risks, depending on whether the cells used in generation of the vector is of human or non-human origin. For AAV, empty capsid, encapsidated host cell DNA, encapsidated helper component DNA, replication competent virus, non-infectious vector, and aggregated, degraded, or oxidized vectors are all considered potential risks.48 Thus, an overall risk assessment followed by a mitigation design should be considered in light of the regulatory standards and patient safety that is required for clinical manufacturing.48
Downstream process challenges
Yield
Yield is an important concern in downstream as loss of viral yield puts increased pressure on the upstream process to increase yield and scale. Viral instability and loss of function can be a huge problem for some vectors such as LV and other enveloped viruses. Reducing the number of steps, and time in process, and reducing shear forces can improve overall yield for unstable vectors. Storing of process intermediates and how infectivity is impacted by the hold conditions (temp, pH, etc.) can also impact yield. Thus, the need to develop a rapid process for unstable viruses is a key consideration in process design.
Purity
Another major challenge in downstream is obtaining sufficient purity without loss of function. For example, in a typical production of an AAV vector there are numerous impurities to consider including host DNA/RNA, host cell protein (HCP), plasmid DNA, helper viruses, culture medium components, items introduced during purifications (buffers, ligands, salts, etc.), empty and partially full capsids, replication competent AAV, noninfectious AAV, and other AAV forms such as aggregates.48 To further complicate matters, residual DNA or host cell protein can adhere to some vectors and co-purify with them. Thus, the removal of impurities is vital as insufficient purity impacts product quality and safety and can ultimately result in negative outcomes with regulatory authorities. In particular, there are concerns over possible immunogenicity and toxicity. In addition, the process must meet regulatory specifications for mycoplasma, adventitious agents, microbial stability, and others.
Overall process design and efficiency is key to controlling impurities. Effort must be taken to avoid introduction of contaminants in the downstream process, unless necessary. Any introduction of contaminants would then require additional analytical and processing steps for their removal. Benzonase treatment can be used to remove residual DNA, however, its use can add cost. Detergents can be used to control enveloped viruses provided the vector virus is non-enveloped. Again, anything that is added to a process must be removed from the final formulation.
For AAV, the process development time for the empty/full capsid separation can be a months-long endeavor. There is no “platform” for empty/full separation and the parameters for empty/full separation by IEC must be carefully developed for each vector. In addition, some AAV vector preparations can enter downstream containing, as much as 90% empty capsids, and developing a process to remove high loads of empty capsids can require significant time and resources. It is helpful to optimize feedstock from upstream so that it contains as many full capsids as possible. Understanding and defining the levels of required exclusion for empty and partially full capsids can aid in the development of a process that meets regulatory requirements.
Areas for advancement
Affinity chromatography
AC shows great promise as it is effective, and its use could potentially enable a platform-like approach in portions of downstream simply by switching AC columns. The advancement of AC media across other viruses and serotypes would be an advancement for the industry. The resins can be expensive, and current protein-based AAV resins cannot be regenerated through many cycles without need to be replaced. Perhaps, in the future, resins could be developed to overcome the regeneration issues and be available at a lower price point.
Automation and closed systems
Automation is needed in several areas to reduce both labor and costs. Closed systems are needed for larger vectors where sterilization by filtration is an issue. Closed systems also serve to protect both the product and the operators and will be of large benefit by several measures. In that regard, more development of processes similar to those that have benefited the recombinant protein industry is needed such as disposable technology and continuous processing methods.
Move toward more efficient methods
The continued movement away from older methods such as ultracentrifugation to more efficient processes such as chromatography will benefit the industry. Nevertheless, much ultracentrifugation is still being performed in the industry, which indicates there are still advancements needed in this area. Alternatives to traditional chromatography resins show great promise to increase efficiency and scale. Moreover, the cost of some specific resins and filters are quite expensive, especially at larger scale. When chromatographic material is packed into highly efficient, single-use columns, process throughput can be further improved and substantial savings in infrastructure, operations and quality procedures can be realized.49
Analytics
On the analytics side, development of better or faster at-line or inline analytics for intermediate crude with lower viral concentration will benefit the industry by enabling process efficiency and lower costs. Because the analytics can be quite imprecise and can take a long time to perform, downstream processing often operates “blind.” High throughput analytics could reduce process development timelines by providing a more comprehensive look at the process and in identifying areas for improvement. For example, analytical ultracentrifugation for AAV, while good, can be expensive and take time but is one of the few methods, together with capillary electrophoresis, that can separate empty from full capsid with good resolution.9,50. Alternate analytics, such as HPLC or transmission electron microscopy (TEM), can sometimes be used inline to reduce costs, or improve throughput. For more information, please see analytics article in this publication.
The development of virus-specific tools
Many tools and technologies currently employed in downstream production have been developed for use with small molecules and antibodies and not for viruses. For example, some resins were developed for the purification of monoclonal antibodies from feedstock containing 5 g/L antibody. Viral vectors are much larger, more complex, and are found in feedstock at 1000-fold less concentration than antibodies. With viruses, column functionality, the capacity to capture, and recovery are much more critical than with monoclonal antibodies. The needs are different.
Other items used at larger scales such as concentration and use of membrane filters, can be entirely different for viruses compared to antibodies. For example, the concentration of 2000L of virus vs 2000L of antibody is very distinct and physical re-engineering of available systems may be helpful. Scale down tools are also needed as many single use platforms were designed for use with 100’s of gram of antibody-compared to viruses at < 1 g for small scale. Thus increased virus-specific tools are needed for both small scale and large scale processing.
Summary
In summary, there is an increasing range of options to process and obtain high titers and high-quality batches of viral vectors. We expect continued advancement and the future is open to new innovations and capabilities that should reduce complexity, lessen the challenges, and lower costs. In many ways, the industry is taking a similar path to the monoclonal antibody industry several years ago with expansive growth and manufacturing capabilities.
Footnotes
- 1. McCarron, A., Donnelley, M. & Parsons, D. Scale-up of lentiviral vectors for gene therapy: advances and challenges. Cell Gene Ther. Insights 3, 719-729 (2017).
- 2. Sommer, J. M. et al. Quantification of adeno-associated virus particles and empty capsids by optical density measurement. Mol Ther 7, 122-128 (2003).
- 3. Merten, O. W. AAV vector production: state of the art developments and remaining challenges. Cell Gene Ther. Insights 2, 521-551 (2016).
- 4. Gao, K. et al. Empty virions In AAV8 vector preparations reduce transduction efficiency and may cause total viral particle dose-limiting side-effects. Mol Ther Methods Clin Dev 1, 20139 (2014).
- 5. Wright, J. F. AAV empty capsids: for better or for worse? Mol Ther 22, 1-2 (2014).
- 6. Carmo, M. et al. Relationship between retroviral vector membrane and vector stability. J Gen Virol 87, 1349-1356 (2006).
- 7. Pall Biotech. Current observation.
- 8. Ausubel, L. J. et al. Production of CGMP-Grade Lentiviral Vectors. Bioprocess Int 10, 32-43 (2012)
- 9. Merten, O. W., Hebben, M. & Bovolenta, C. Production of lentiviral vectors. Mol Ther Methods Clin Dev 3, 16017 (2016).
- 10. White, M., Whittaker, R., Gandara, C. & Stoll, E. A. A guide to approaching regulatory considerations for lentiviral-mediated gene therapies. Hum Gene Ther Methods 28, 163-176 (2017).
- 11. Benchaouir, R. Recent advances in the purfication of adeno-associated virus. Cell Gene Ther. Insights 2, 287-297 (2016).
- 12. Dias Florencio, G. et al. Simple downstream process based on detergent treatment improves yield and in vivo transduction efficacy of adeno-associated virus vectors. Mol Ther Methods Clin Dev 2, 15024 (2015).
- 13. CRS. CRS-Chemical inspection and regulation service -REACH Restriction. Accessed Retrieved from http://www.cirs-reach.com/REACH/REACH_Restriction.html
- 14. GE Healthcare (2018). Application Note: Optimization of midstream cell lysis and virus filtration steps in an adenovirus purification process. Accessed Oct. 20, 2020: Retrieved from https://cdn.cytivalifesciences.com/dmm3bwsv3/AssetStream.aspx?mediaformatid= 10061&destinationid=10016&assetid=26781
- 15. Merten, O. W. et al. Large-scale manufacture and characterization of a lentiviral vector produced for clinical ex vivo gene therapy application. Hum Gene Ther 22, 343-356 (2011)
- 16. Geraerts, M., Michiels, M., Baekelandt, V., Debyser, Z. & Gijsbers, R. Upscaling of lentiviral vector production by tangential flow filtration. J Gene Med 7, 1299-1310 (2005).
- 17. Cooper, A. R. et al. Highly efficient large-scale lentiviral vector concentration by tandem tangential flow filtration. J Virol Methods 177, 1-9 (2011).
- 18. Potter, M., Chesnut, K., Muzyczka, N., Flotte, T. & Zolotukhin, S. Streamlined large-scale production of recombinant adeno-associated virus (rAAV) vectors. Methods Enzymol 346, 413-430 (2002).
- 19. Zhao, M. et al. Affinity chromatography for vaccines manufacturing: Finally ready for prime time? Vaccine (2018).
- 20. Silva, R., Mota, J., Peixoto, C., Alves, P. & Carrondo, M. Improving the downstream processing of vaccine and gene therapy vectors. Pharm. Bioprocess. 3, 489-505 (2015).
- 21. Pall Biotech. Why membrane adsorbers? Accessed Oct. 3, 2020: Retrieved from https://pall.com/en/biotech/chromatography/membrane-chromatography.html
- 22. all, P. & Gyepi-Garbrah, I. Membrane adsorbers as a tool for rapid purification of gene therapy products. Accessed Oct. 9, 2020: Retrieved from https://www.pall.com/content/dam/pall/laboratory/literature-library/non-gated/Mustang_poster_841x1189mm.pdf
- 23. Summerford, C. & Samulski, R. J. Viral receptors and vector purification: new approaches for generating clinical-grade reagents. Nat Med 5, 587-588 (1999).
- 24. Segura, M. M., Kamen, A., Trudel, P. & Garnier, A. A novel purification strategy for retrovirus gene therapy vectors using heparin affinity chromatography. Biotechnol Bioeng 90, 391-404 (2005).
- 25. Mietzsch, M., Broecker, F., Reinhardt, A., Seeberger, P. H. & Heilbronn, R. Differential adeno-associated virus serotype-specific interaction patterns with synthetic heparins and other glycans. J Virol 88, 2991-3003 (2014).
- 26. Kanlaya, R. & Thongboonkerd, V. Cellufine sulfate column chromatography as a simple, rapid, and effective method to purify dengue virus. J Virol Methods 234, 174-177 (2016).
- 27. GE Healthcare. AVB Sepharose™ High Performance. Data file 28-9207-54 AB.
- 28. Nass, S. A. et al. Universal Method for the Purification of Recombinant AAV Vectors of Differing Serotypes. Mol Ther Methods Clin Dev 9, 33-46 (2018).
- 29. Mietzsch, M. et al. OneBac: platform for scalable and high-titer production of adeno-associated virus serotype 1-12 vectors for gene therapy. Hum Gene Ther 25, 212-222 (2014).
- 30. Thermo Fisher. Accessed Retrieved from http://thermofisher.mediaroom.com/press-releases?item=122399
- 31. Scherr, M. et al. Efficient gene transfer into the CNS by lentiviral vectors purified by anion exchange chromatography. Gene Ther 9, 1708-1714 (2002).
- 32. Khandelwal, P. (2015). (Bulletin 6807)Practical guide: selecting theoptimal resins for adenovirus process purificatioin. Accessed Oct. 21, 2020: Retrieved from https://www.bio-rad.com/webroot/web/pdf/psd/literature/Bulletin_6807.pdf
- 33. Wright, J. F. & Zelenaia, O. Vector characterization methods for quality control testing of recombinant adeno-associated viruses. Methods Mol Biol 737, 247-278 (2011).
- 34. Manno, C. S. et al. Successful transduction of liver in hemophilia by AAV-Factor IX and limitations imposed by the host immune response. Nat Med 12, 342-347 (2006).
- 35. Okada, T. et al. Scalable purification of adeno-associated virus serotype 1 (AAV1) and AAV8 vectors, using dual ion-exchange adsorptive membranes. Hum Gene Ther 20, 1013-1021 (2009).
- 36. Urabe, M. et al. Removal of empty capsids from type 1 adeno-associated virus vector stocks by anion-exchange chromatography potentiates transgene expression. Mol Ther 13, 823-828 (2006).
- 37. Davidoff, A. M. et al. Purification of recombinant adeno-associated virus type 8 vectors by ion exchange chromatography generates clinical grade vector stock. J Virol Methods 121, 209-215 (2004).
- 38. Wang, C. et al. Developing an anion exchange chromatography assay for determining empty and full capsid contents in AAV6.2. Mol Ther Methods Clin Dev 15, 257-263 (2019).
- 39. Rodrigues, T., Carrondo, M. J., Alves, P. M. & Cruz, P. E. Purification of retroviral vectors for clinical application: biological implications and technological challenges. J Biotechnol 127, 520-541 (2007).
- 40. Transfiguracion, J., Jaalouk, D. E., Ghani, K., Galipeau, J. & Kamen, A. Size-exclusion chromatography purification of high-titer vesicular stomatitis virus G glycoprotein-pseudotyped retrovectors for cell and gene therapy applications. Hum Gene Ther 14, 1139-1153 (2003).
- 41. Shambram, P., Vellekamp, G. & Scandella, C. in Adenoviral vectors for gene therapy (Academic Press, 2016).
- 42. Chahal, P. S., Aucoin, M. G. & Kamen, A. Primary recovery and chromatographic purification of adeno-associated virus type 2 produced by baculovirus/insect cell system. J Virol Methods 139, 61-70 (2007).
- 43. Burns, J. C., Friedmann, T., Driever, W., Burrascano, M. & Yee, J. K. Vesicular stomatitis virus G glycoprotein pseudotyped retroviral vectors: concentration to very high titer and efficient gene transfer into mammalian and nonmammalian cells. Proc Natl Acad Sci U S A 90, 8033-8037 (1993).
- 44. Jiang, W. et al. An optimized method for high-titer lentivirus preparations without ultracentrifugation. Sci Rep 5, 13875 (2015).
- 45. Buclez, P. O. et al. Rapid, scalable, and low-cost purification of recombinant adeno-associated virus produced by baculovirus expression vector system. Mol Ther Methods Clin Dev 3, 16035 (2016).
- 46. Slepushkin, V. et al. Large-scale purification of a lentiviral vector by size exclusion chromatography or Mustang Q ion exchange capsule. Bioproc J 2, 89-95 (2003).
- 47. Hernandez, R. (Apr. 1, 2015). Continuous manufacturing: A changing processing paradigm. Accessed Oct, 22, 2020: Retrieved from https://www.biopharminternational.com/view/continuous-manufacturing-changing-processing-paradigm
- 48. Wright, J. F. Product-related impurities in clinical-grade recombinant AAV vectors: characterization and risk assessment. Biomedicines 2, 80-97 (2014).
- 49. Jacquemart, R. et al. A Single-use Strategy to Enable Manufacturing of Affordable Biologics. Comput Struct Biotechnol J 14, 309-318 (2016).
- 50. Zolotukhin, S. et al. Recombinant adeno-associated virus purification using novel methods improves infectious titer and yield. Gene Ther 6, 973-985 (1999).