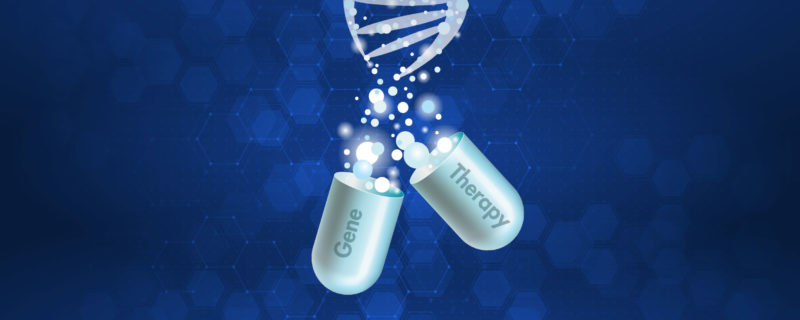
Downstream Manufacturing of Gene Therapy Vectors
Introduction
The goal of downstream processing is to separate the viral vector from the various impurities produced during upstream processing and to get the virus into the appropriate state for formulation and administration to patients. Viral vector batches destined for clinical use must comply to increasing regulatory standards for impurities and contaminants as these can affect product safety and potency. In addition to providing purity, purification processes need to meet production scale, which can be quite large for clinical trials and commercial applications.
Approaches to vector purification exploit the physical characteristics of the viral particle such as size, surface charge, and hydrophobicity. Viral vectors vary greatly in physical characteristics depending on the type of virus, the serotype of the same virus, and can sometimes vary with different transgene inserts within the same vector (AAV). Table 1 shows some similarities and differences of the 4 commonly used viruses used as vectors: adenovirus (AdV), adeno-associated virus (AAV), retrovirus (RV), and lentivirus (LV).
Virus | Size (nm) | Envelope | Stability | Buoyant Density |
---|---|---|---|---|
Adenovirus | ~90 | No | High | 1.34 (CsCl) |
AAV | ~ 20 | No | High | 1.41 (CsCl) |
Retrovirus/Lentivirus | ~90-120 | Yes | Low* | 1.16 (Sucrose) |
a. Pseudotyping with VSV-g is reported to improve stability to some extent1
The differences in physical characteristics between various vectors presents a diverse set of purification challenges that result in a multiplicity of needed techniques and solutions for the industry. For example, AAV also accumulated in the media and is partly released from cells via a lytic process, AAV viruses are generally harvested by cell lysis to improve yield. Cell lysis can generate significant quantities of host cell contaminants including DNA and protein that increase purification burden. AAV vectors can produce a significant portion of “empty” particles devoid of transgene at up to 95% to total particles.2 Despite the ongoing debate over a possible benefit from the presence of empty particles in AAV therapies, these are still considered a major contaminant3,4 and health authorities encourage sponsors to set limits on the amount of empty or partially full vectors.
The downstream processing of RV/LV vectors presents its own unique challenges. The presence of a lipid envelope makes these vectors less stable. The instability of these particles can lead to a loss of yield – potentially up to 70% during the whole downstream purification process.5 Pseudotyping can also influence RV/LV stability, which may be improved or made worse depending on the vector and pseudotype component.1,6 Thus, each vector can present unique challenges during downstream processing.
There are other unique challenges for the purification of vectors that are generated because of the chosen upstream production process. For example, vector supernatants that are produced by transfection contain large amounts of contaminating plasmid DNA. Large viruses, such as herpes, may not be filter sterilizable, and may requiring aseptic processing, and the validation that goes with that.7,8,9 The use of serum-supplemented cell culture media can result in a greater purification burden. Moreover, increased viscosity from the use of sera can affect downstream process efficiency.10 In this regard, upstream production systems using serum-free media, such as common with cells grown in bioreactors and with baculovirus/insect cell systems, can produce a significant advantage downstream.
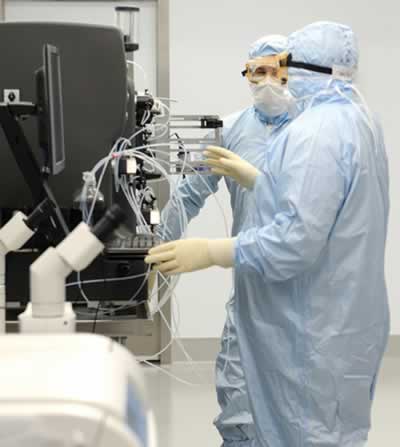
Despite the differences in physical characteristics between vectors, current downstream protocols generally have a similar work flow which involve a clarification step (which may be proceeded by cell lysis), purification (centrifugation or ion exchange/affinity chromatography), and a polishing step (additional chromatography step/size exclusion) (Figure 1). In addition to the above, filtration/dialysis/centrifugations steps are typically utilized to concentrate, for buffer exchange, or for final formulation.
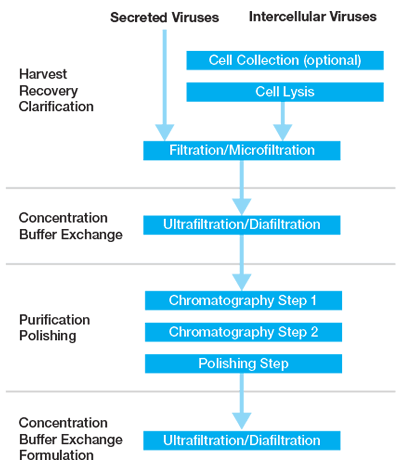
Clarification, Cell Lysis, and Filtration
The clarification step includes elimination of large debris and macromolecular complexes from the initial crude suspension and may include cell lysis in order to improve viral yield (AdV, AAV). Physical methods such as microfluidizers or heat-shock treatments can be used to promote cell lysis. Lysis using detergents such Triton™ X-100 or other detergents has proven inexpensive and efficient for many applications such as for AdV and AAV preparations and can be utilized for insect cell preparations as well.11 However, the inclusion of Triton™ X-100 on the REACH list of banned substances in the EU limits its adoption.12 Alternative approaches are available using Tween™ detergents13 and there are analytical methods for the detection of Tween™ 20 in vector preparations. However, polysorbate 20/80 are thought to adversely impact chromatographic steps, reducing the dynamic binding capacity and fouling the underlying chemistry of the resin/ligand interactions.
During or after the cell lysis step, nucleases (example benzonase) are typically added in order to degrade nucleic acids and disturb the macromolecular complexes. Any cell lysis method requires optimization as lysis is highly dependent on the cell concentration, incubation time, incubation temperature, cell type, and the virus being released.15
The initial removal of cell debris and impurities during clarification typically involve a combination of filters with decreasing pore size down to 0.2 µm, which act as an important part of an overall bioburden control strategy. Note that clarification of larger viruses, may be limited to larger pore sizes due to possible retention of virus or issues with excessive shear force. Diafiltration can also prove effective for clarification in addition to serving other functions such as concentration and buffer exchange. A key consideration for this unit operation is the inclusion of a pre-filter with relatively large nominal pore sizes to remove cellular debris and protect subsequent filters from clogging.
During the processing of RV/LV, reduced viral yield due to the low stability of particles is an upmost concern. Process strategies may be need to be developed to minimize shear such as limiting flow rates or employing the use of slower speed centrifugation. At smaller scale, slow speed centrifugation can provide some degree of clarification for RV/LV, while at larger scale direct flow setups have been used with some vectors using 1 µm > 0.8 µm > 0.45 µm filters.14 Alternatively, tangential flow filtration (TFF) has proven an effective method for both clarification and concentration with reports of recoveries as high as 90–100%, although flow rate may need to be adjusted.15,16 Exploration of single-pass TFF systems is an active area of development given the potential to improve processing yields while minimizing potential shear forces.
The Limitation of Classical Centrifuge Methods
Purification of small quantities of virus for research or for small clinical studies have classically relied heavily on ultracentrifugation methods. In these methods, viral particles are typically pelleted and concentrated through a matrix, which is then followed by resuspension and purification via buoyant density using gradient ultracentrifugation. Several rounds of gradient ultracentrifugation may be required to further purify particles (Table 1).
CsCl has been commonly used for AAV and AdV ultracentrifugation in the past. However, iodixanol is now preferred over CsCl because it is less detrimental, needs less dialysis, lessens aggregation, and because run times are shortened from 24 h to few hours.17 Because of the decreased stability of enveloped viruses such as RV/LV, sucrose is generally used for density purification of these. For example, classical retroviral techniques using ultracentrifugation typically involve concentration by “pelleting“ particles through a cushion of 20% sucrose, resuspending, and then “banding” by sucrose gradient ultracentrifugation(s).
A major problem with density methods is scale up due to centrifuge volume limitations and cost. Since larger volume ultracentrifuges are now available on the market, it is now possible to at least consider ultracentrifugation at some scales. However, any benefit must be weighed against the disadvantages of labor, processing time, possible capex, and other restraints.10 In addition, there are health and safety issues associated with density ultracentrifugation as recovery of the vector usually requires sticking a needle into the side of the ultracentrifugation tube, which certainly increases safety concerns for using sharps with highly concentrated vectors.
Despite these and other drawbacks, ultracentrifugation has been adopted by a number of companies and academic institutions to produce early-stage clinical trial material. Some companies have shown ultracentrifugation has application in the later stages of an overall purification scheme and can in some instances satisfy most of the purification needs at smaller scale.10 Moreover, buoyant density separation of some viruses such as AAV has clear benefits as it is serotype independent and it is one of the few methods that can separate full AAV particles from empty particles. Moreover, density ultracentrifugation can separate helper AdV from full AAV particles.8,18
Chromatography
The use of chromatography for the purification of vectors has advanced and is becoming an established method that can purify vector by its physical property of net charge, hydrophobicity, affinity to ligand, size, or other property. Chromatography is considered much more scalable and cost effective than centrifugation.19 Columns can be reused multiple times and columns can be run in parallel or in tandem in different strategies. In addition, chromatography is an effective means of removing potential adventitious agents, a key aspect of late-stage development. Chromatography has been widely used for purification of vaccines and gene therapy vectors and it may be applied in various downstream steps, including capture, concentration, purification, and polishing steps.20
Ion Exchange Chromatography
Ion exchange chromatography (IEC) is based on surface charge and is a simple and cost-effective technique that can be applied to multiple virus types. Anion or cation exchangers can be used to bind either positively or negatively charged viruses. However, IEC requires developmental efforts to match the column type to individual virus surface properties, such as found in varying serotypes. One IEC step may not be sufficient to purify some viruses such as AAV, which then requires additional processing steps.21
The use of IEC can have clear benefits. IEC is versatile. For example, with AAV vectors, IEC can work across multiple serotypes and can also be used to separate helper viruses such as AdV and baculovirus from AAV preparations.22 IEC is one of the few methods that can separate full from empty AAV particles due to a difference in the charge of full particles from the presence of full-length DNA.23 IEC can also be effective in this regard as protocols have shown >90% elimination of empty particles.23,24,25 Examples include a 2-stage process where cation exchange (Mustang® S, Pall Corp.) was used to retain empty particles, which is followed by strong anion exchange (Mustang® Q) to retain full particles. This process resulted in only 0.8% empty particles.23
IEC theoretically should allow the separation of empty particles of all AAV serotypes; however, resins and conditions would likely need to be optimized for each individual serotype.21,26 One drawback to the separation of empty particles is that substantial development time (>2 months) may be required as the gradients necessary for separation can be very shallow. Furthermore, anything that affects the charge of a vector, such as serotype, vector design, or length of the transgene insert, may require redevelopment of the gradient conditions.
IEC is a promising purification method. Currently, at larger scale, the only efficient way to separate full from empty particles is by use of ion-exchange chromatography.3 There are many configurations available that include resins, faster flow membranes, such as Mustang®, or other configurations. IEC is flexible, such as being used as a capture step, which then can be followed by a buoyant density ultracentrifugation. This method has been used for the generation of GMP AAV vector for multiple clinical studies.3,27,28 In addition, IEC can produce LV vectors of high purity, although the use of too high of ionic strength solutions during the elution step can reduce LV infectivity.29
Hydrophobic Interaction Chromatography
Hydrophobic interaction chromatography (HIC) been used for viral capture/clearance in the vector and recombinant protein industry for years. In purification schemes, HIC has been used for the purification both AdV and AAV2.30, 31 However, its use with AAV has not been extensively documented with different serotypes. Similar, to IEC use of high ionic strength solutions during binding can affect the stability of some viruses.30
Affinity Chromatography
Affinity chromatography (AC) relies on the interaction of the viral particle with a ligand. Heparin affinity chromatography has been used for purification of LV and other viruses and is also reported to handle large volumes.32 The use of mild salt solutions and can help preserve vector integrity.33 However, there are disadvantages including non-specific binding of impurities that then require additional purification steps32, animal sourcing, and selectivity of only some AAV serotypes such as 2, 3, 6, and 13. 34 Cellufine™ sulphated cellulose is an alternative with similar specificity to heparin affinity for purification of various viruses.35
For AAV, AVB affinity resin (BAC/GE Healthcare) has been shown to be effective strategy for the purification of AAV serotypes 1, 2, 3, and 5 as recommended by the manufacturer.36 Others have reported its successful use with other serotypes, although efficiency may vary depending serotype.37,38 Moreover, an analytical kit is available to check for leakage of the recombinant protein ligand.39 AVB has successfully been used in combination with IEC to purify a variety of AAV serotypes.37
Newer serotype-specific affinity matrices are available for AAV serotypes 8 and 9 (Thermo Fisher, POROS CaptureSelect™). These have resulted in the development efficient vector-specific purification protocols that consist of clarification, affinity chromatography, tangential flow filtration, and a gel filtration step for formulation.3,19 These affinity matrices reportedly also lead to an increase in the overall yield3, and a lower cost of goods.40 Genethon has reported purification of AAV9 using CaptureSelect™ AAV9 at 10L and 50L scale with >80% recovery.19
One issue with the use of affinity chromatography with AAV is that full particles cannot be separated from empty particles, which results in additional steps such as ion-exchange chromatography or ultracentrifugation for that purpose.37 Another potential issue with affinity chromatography is the possible leakage of ligand into the vector preparation. Leakage of ligand may require analytical steps and possible additional purification steps.
Size Exclusion Chromatography
Size exclusion chromatography (SEC) separates virus particles from contaminants on the basis of size and mass.41 SEC has benefits in that it is very gentle on particles since there is no binding or elution. However, SEC may not be suitable for large-scale processing as it has low throughput, requires low flow rates that increase processing time, and it may dilute the sample and result in additional concentration steps. Moreover, it is commonly thought that SEC does not scale well beyond 1000L. Nevertheless, SEC has found use as a final polishing for many viruses including AAV and LV.8,41,42
Concentration/Buffer Exchange
Pelleting virus by ultracentrifugation can provide effective concentrations and high vector yields for some vectors. However, pelleting has potential disadvantages such as losses of functional vector particles due to shear stress43 and co-concentration of impurities.42 The use of low-speed centrifugation for longer durations is a more gentle approach, which may prove valuable with less stable vectors to result in higher infectious particle recoveries.44 However, as discussed above centrifugation methods are judged to have limited scalability.
TFF has advantages for concentration or buffer exchange in that it is typically scalable and allows for mild processing conditions for less stable vectors. Products are available in numerous configurations based on the molecular weight cutoff, the nature of the membranes, or the filter surface. Although the achievable concentration factors are often lower than for centrifugation methods there is an additional benefit in that some impurities may be removed during the process of concentration.17,45
Bioburden Control
Bioburden control is critical for products with a GMP regulatory requirement to offset the risk of microbial contamination of the final product. In most protocols, the final vector preparation is filtered by 0.2 µm filtration as component of control, although there may be variations in its placement in downstream protocols.3 Some vector products are not filtered sterilizable because of the size of the vector. It is possible to skip sterile filtration provided that the process can be certified as being fully aseptic; however, this requires validation and operations must be performed in a clean room. Nevertheless, there are large-scale protocols without the sterile filtration step because of the use of a semi-closed system.7 TFF systems can provide increased throughput for sterilization and can have benefit in that regard.
Moving Downstream from Research Production to Clinical/Commercial Manufacturing
One of the major considerations of moving to clinical manufacturing is how to employ increased production scale. At larger scales, the purification methods need to accommodate large volumes with high efficiency and speed. One approach toward implementation of greater scale is to move away from poorly scalable centrifugation-based techniques to systems with greater throughput. Chromatographic approaches are considered to be the best technological method for manufacturing applications, due in part to increased scalability. Moreover, IEC or AC methods can be used as starting methods for the purification of many vectors.10,23 When considering individual chromatography, efficiency is a huge factor that can lead to a smaller, simpler process and greater scale.
It has been shown that VSG-g LV vectors can be purified using membrane based Mustang® Q capsules (Pall Corp.) up to a volume of 1,500 L/day46 and lead to vector preparations of high purity. However, LV recovery may not be as efficient as with ultracentrifugation due to the fragility of these vectors. In this case, there may a clear trade off between efficiency and yield.
Another major aspect is GMP compliance. Vectors for therapeutic use must meet stringent standards for purity and safety. In this regard, product quality is of upmost importance. Simplification of the process design and increase of efficiency can reduce the number of required costly GMP reagents and steps, which significantly lower costs. Adventitious agent control is an aspect of GMP that is only generally considered once a process has moved into clinical production. Adventitious virus control is a particular challenge as conventional virus control techniques developed for mAb production, such as the viral inactivation step or the viral removal step, may not be suitable when a virus is the product of interest. Closed processing can be helpful for agent control; however, many remain under development by the industry. Moreover, development of a closed system requires working closely with vendors to ensure that a fully closed flow path is designed and is suitable for the process.
Challenges in Downstream
There are always challenges with development of a downstream process. Yield is of paramount important. Loss of viral yield puts pressure on increased pressure on the upstream process for greater titer. Viral stability can be a huge problem for some vectors. Reducing the number of steps and time in process can support viral stability and improve overall yield. Enveloped vectors and some pseudotyped vectors can be especially unstable and conditions that reduce shear forces can help with yield. Storing of process intermediates and how infectivity is impacted by the hold conditions (temp, pH, etc.) is a major challenge.
Caution must taken be for the introduction of contaminations in the downstream process. The use of lysis buffers, affinity resins, and other methods can introduce contaminants, which would then require the need for analytical assays and the possibility of additional processing steps.
Aggregation can be issue during downstream processing particularly when viruses are released by lysis or when concentrated. Some methods are prone to producing aggregates, such as concentration by ultracentrifugation pelleting. Choosing alternate methods or conditions can help reduce aggregation.
Cost Considerations in Downstream Manufacturing
Cost is a combination of many factors such as, time, labor, operating expenses, and capital expenses. The technology used in downstream processing can impact the timeline of process development, product quality, ease of process scale-up, and cost of goods (COGs).19 Many of the traditional methods of viral purification are time consuming, complex, have low productivity, are not efficient, and can add substantially to cost.20 Replacing traditional methods with approaches that are more cost effective, such as chromatography, can lower costs.
Implementing newer, more efficient strategies such as IEC or AC that have greater potential for throughput and upscaling can significantly simplify process strategy and increase efficiency. These simple and potentially platformable vaccine or vector purification schemes significantly shorten process development time and reduce COGs. In that regard, the newer affinity chromatography approaches, if applicable to the particular vector, can potentially simplify the process architecture to a single chromatography capture step accompanied only by a clarification step and a polishing step. The use of purification and polishing chromatographic systems can provide quality vectors of high purity as well.10
Development and use of lower costs structures such as (semi)-continuous processes systems can result in footprint reduction, increased productivity, automations, reduced inventory and storage, and fewer, unit operations, can reduce costs.20,47 Reduction of the chromatographic unit operations, such as multi-column systems, and single-use systems can also reduce cost can cost.20 An additional chromatographic approach to reduce costs is by employing column cycling with expensive resins. When implementing scalable solutions for downstream, such as depth filtration, chromatography etc. it is possible to reduce processing time multiple-fold as well as the process being scalable.
Enabling the use of tangential flow filtration (TFF), which can be scaled up during manufacturing can provide cost savings. In that regard, automated TFF systems are available to control both the transmembrane pressure as well as the level of concentration. Additionally, automation of TFF can provide an ergonomic and secure environment for the operator and facilitate the speed and the upscaling of the process. A 20–80x concentration factor can easily be achieved using these systems.10
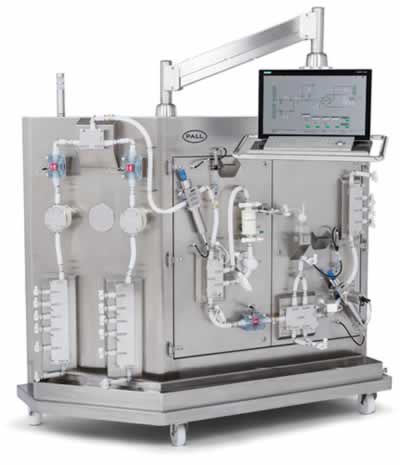
Areas for Improvement
With respect with downstream there are several areas that need improvement or are in the process of being improved by the industry. Implementation of automation could be a big advancement. Automation is needed in several areas to reduce both labor and costs. Closed systems are needed for larger vectors that where sterilization by filtration is an issue. Closed systems also serve to protect both the product and the operators and will be of large benefit by several measures. In that regard, more development of processes similar to those that have benefited the recombinant protein industry is needed such as disposable technology, and continuous processing methods.
The continued movement away from older methods such as ultracentrifugation to more efficient processes such as chromatography will benefit the industry. Alternatives to traditional chromatography resins that incorporate improved designs for viruses such as nanofiber and macroporous filters show great promise to increase efficiency and scale. Moreover, the costs of some specific resins and filters are quite expensive and could be challenging during the launch of a clinical project3 Some affinity resins are very expensive.
There continues to be a challenge with upscaling. Continued development of upscaling methods through the 2000L scale would benefit the industry greatly. There is some upscaling struggle for empty vs. full AAV separation and there are still several issues that need solutions. For example, volumes of buffers and fractionation could be an issue if the elution gradient is long.
On the analytics side, implementation of at-line or inline analytics will benefit the industry by allowing more efficient time in process and lowering costs. Because the analytics can be quite imprecise and can take a long time to perform, downstream processing can take place “blind”. One of the results of this is that recovery from a downstream process can be very low with some vectors (between 5 – 30%). Another challenge with the analytics is achieving high throughput, which limits efficiency and can also extend development time.
Establishing efficient downstream protocols for LVs has proven challenging. Loss of yield due to virus stability is a crucial bottleneck with the manufacturing of many vectors. As a result, purification and concentration techniques have been often integrated together to create a step-wise downstream approach to minimize losses. Further improvements to enhance yield of less stable vectors, such as low shear systems, new handing methods, and stabilization methods will benefit the industry.
AC shows great promise as it could potentially enable a platform-like approach where a universal downstream scheme is suitable for purifying multiple products simply by switching AC columns. When chromatographic material is packed into highly efficient, single-use columns, process throughput can be further improved and substantial savings in infrastructures, operations and quality procedures can be realized.48
One drawback is that approaches that rely on particle charge or affinity to ligand, can be virus/serotype dependent, meaning that a major technological development must be undertaken for each AAV serotype. In addition, it is currently very difficult to separate the full and empty particles using these approaches.
Process Development Timeline
The timeline to develop a downstream process is dependent on many factors such as the type of vector, the intended use, the scale, cost considerations, and the technology available. Independent of the factors involved, reduced developmental timelines are a desirable goal. Moreover, reduced timelines lower cost. Timelines for scientific work on a downstream process for complex molecule can be extensive – several months – depending on the resources available. Technical transfer can add additional time.
One promising approach is that technology is advancing for some vectors that is enabling more “platform-like” or “plug and play” solutions for downstream. This is particularly being seen in IEC and AC chromatography. For example: most AdV 5 vectors should have similar surface properties. In that case, developmental timeline for various AdV 5 vectors could be reduced significantly since only tweaking of the process is needed. Similarly for AAV, the rise of vector-specific AC solutions could potently reduce developmental timelines in that columns can be switched for vectors of different serotype.19
Summary
In summary, there is an increasing range of options to process and obtain high titers and high-quality batches of viral vectors. These new developments, particularly in the chromatography approaches, have improved downstream process options, which have in turn enabled gene therapy. There are much needed improvements, especially in the areas of upscaling and lowering cost. Nevertheless, the industry is advancing, and the future is open to new innovations and capabilities.
This article is the third in a series that was published in our eBook, “Key Considerations for Gene Therapy Manufacturing for Commercialization“. You can download all the articles in the series, by downloading the eBook below.
Footnotes
-
1. McCarron, A., M. Donnelley, and D. Parsons, Scale-up of lentiviral vectors for gene therapy: advances and challenges. Cell GeneTherapy Insights, 2017. 3(9): p. 719-29.
-
2. Sommer, J.M., et al., Quantification of adeno-associated virus particles and empty capsids by optical density measurement. Mol Ther, 2003. 7(1): p. 122-8.
-
3. Merten, O.W., AAV vector production: state of the art developments and remaining challenges. Cell GeneTherapy Insights, 2016. 2(5): p. 521-551.
-
4. Gao, K., et al., Empty Virions In AAV8 vector preparations reduce transduction efficiency and may cause total viral particle dose-limiting side-effects. Mol Ther Methods Clin Dev, 2014. 1(9): p. 20139.
-
5. Carmo, M., et al., Relationship between retroviral vector membrane and vector stability. J Gen Virol, 2006. 87(Pt 5): p. 1349-56.
-
6. Tailor, C., Challenges and advances in viral vectors. Cell Gene Therapy Insights, 2016. 2(5): p. 583-588.
-
7. Ausubel, L.J., et al., Production of CGMP-Grade Lentiviral Vectors. Bioprocess Int, 2012. 10(2): p. 32-43.
-
8. Merten, O.W., M. Hebben, and C. Bovolenta, Production of lentiviral vectors. Mol Ther Methods Clin Dev, 2016. 3: p. 16017.
-
9. White, M., et al., A guide to approaching regulatory considerations for lentiviral-mediated gene therapies. Hum Gene Ther Methods, 2017. 28(4): p. 163-176.
-
10. Benchaouir, R., Recent advances in the purfication of adeno-associated virus. Cell GeneTherapy Insights, 2016. 2(3): p. 287-297.
-
11. Dias Florencio, G., et al., Simple downstream process based on detergent treatment improves yield and in vivo transduction efficacy of adeno-associated virus vectors. Mol Ther Methods Clin Dev, 2015. 2: p. 15024.
-
12. CRS. CRS-Chemical inspection and regulation service -REACH Restriction. 2018; Available from: cirs-reach.com/REACH/REACH_Restriction.html.
-
13. Healthcare, G., Application Note: Optimization of midstream cell lysis and virus filtration steps in an adenovirus purification process. 2018.
-
14. Merten, O.W., et al., Large-scale manufacture and characterization of a lentiviral vector produced for clinical ex vivo gene therapy application. Hum Gene Ther, 2011. 22(3): p. 343-56.
-
15. Geraerts, M., et al., Upscaling of lentiviral vector production by tangential flow filtration. J Gene Med, 2005. 7(10): p. 1299-310.
-
16. Cooper, A.R., et al., Highly efficient large-scale lentiviral vector concentration by tandem tangential flow filtration. J Virol Methods, 2011. 177(1): p. 1-9.
-
17. Potter, M., et al., Streamlined large-scale production of recombinant adeno-associated virus (rAAV) vectors. Methods Enzymol, 2002. 346: p. 413-30.
-
18.
- Zolotukhin, S., et al., Recombinant adeno-associated virus purification using novel methods improves infectious titer and yield. Gene Ther, 1999. 6(6): p. 973-85.
- Zhao, M., et al., Affinity chromatography for vaccines manufacturing: Finally ready for prime time? Vaccine, 2018.
- Silva, R., et al., Improving the downstream processing of vaccine and gene therapy vectors. Pharm. Bioprocess., 2015. 3(8): p. 489-505.
- Merten, O.W., et al., Manufacturing of viral vectors for gene therapy: part I. Upstream processing. Pharm. Bioprocess., 2014. 2(2): p. 182-203.
- Khandelwal, P., Practical guide: selecting theoptimal resins for adenovirus process purificatioin, Bio-Rad, Editor. 2016.
- Okada, T., et al., Scalable purification of adeno-associated virus serotype 1 (AAV1) and AAV8 vectors, using dual ion-exchange adsorptive membranes. Hum Gene Ther, 2009. 20(9): p. 1013-21.
- Urabe, M., et al., Removal of empty capsids from type 1 adeno-associated virus vector stocks by anion-exchange chromatography potentiates transgene expression. Mol Ther, 2006. 13(4): p. 823-8.
- Davidoff, A.M., et al., Purification of recombinant adeno-associated virus type 8 vectors by ion exchange chromatography generates clinical grade vector stock. J Virol Methods, 2004. 121(2): p. 209-15.
- Qu, G., et al., Separation of adeno-associated virus type 2 empty particles from genome containing vectors by anion-exchange column chromatography. J Virol Methods, 2007. 140(1-2): p. 183-92.
- Wright, J.F. and O. Zelenaia, Vector characterization methods for quality control testing of recombinant adeno-associated viruses. Methods Mol Biol, 2011. 737: p. 247-78.
- Manno, C.S., et al., Successful transduction of liver in hemophilia by AAV-Factor IX and limitations imposed by the host immune response. Nat Med, 2006. 12(3): p. 342-7.
- Scherr, M., et al., Efficient gene transfer into the CNS by lentiviral vectors purified by anion exchange chromatography. Gene Ther, 2002. 9(24): p. 1708-14.
- Shambram, P., G. Vellekamp, and C. Scandella, Purification of adenovirus, in Adenoviral vectors for gene therapy 2016, Academic Press.
- Chahal, P.S., M.G. Aucoin, and A. Kamen, Primary recovery and chromatographic purification of adeno-associated virus type 2 produced by baculovirus/insect cell system. J Virol Methods, 2007. 139(1): p. 61-70.
- Summerford, C. and R.J. Samulski, Viral receptors and vector purification: new approaches for generating clinical-grade reagents. Nat Med, 1999. 5(5): p. 587-8.
- Segura, M.M., et al., A novel purification strategy for retrovirus gene therapy vectors using heparin affinity chromatography. Biotechnol Bioeng, 2005. 90(4): p. 391-404.
- Mietzsch, M., et al., Differential adeno-associated virus serotype-specific interaction patterns with synthetic heparins and other glycans. J Virol, 2014. 88(5): p. 2991-3003.
- Kanlaya, R. and V. Thongboonkerd, Cellufine sulfate column chromatography as a simple, rapid, and effective method to purify dengue virus. J Virol Methods, 2016. 234: p. 174-7.
- Healthcare, G., AVB Sepharose™ High Performance. Data file 28-9207-54 AB.
- Nass, S.A., et al., Universal Method for the Purification of Recombinant AAV Vectors of Differing Serotypes. Mol Ther Methods Clin Dev, 2018. 9: p. 33-46.
- Mietzsch, M., et al., OneBac: platform for scalable and high-titer production of adeno-associated virus serotype 1-12 vectors for gene therapy. Hum Gene Ther, 2014. 25(3): p. 212-22.
- Fisher, T., CaptureSelect™ AVB Sepharose HP Ligand Leakage ELISA.
- Fisher, T. 2017; Available from: thermofisher.mediaroom.com/press-releases?item=122399.
- Rodrigues, T., et al., Purification of retroviral vectors for clinical application: biological implications and technological challenges. J Biotechnol, 2007. 127(3): p. 520-41.
- Transfiguracion, J., et al., Size-exclusion chromatography purification of high-titer vesicular stomatitis virus G glycoprotein-pseudotyped retrovectors for cell and gene therapy applications. Hum Gene Ther, 2003. 14(12): p. 1139-53.
- Burns, J.C., et al., Vesicular stomatitis virus G glycoprotein pseudotyped retroviral vectors: concentration to very high titer and efficient gene transfer into mammalian and nonmammalian cells. Proc Natl Acad Sci U S A, 1993. 90(17): p. 8033-7.
- Jiang, W., et al., An optimized method for high-titer lentivirus preparations without ultracentrifugation. Sci Rep, 2015. 5: p. 13875.
- Buclez, P.O., et al., Rapid, scalable, and low-cost purification of recombinant adeno-associated virus produced by baculovirus expression vector system. Mol Ther Methods Clin Dev, 2016. 3: p. 16035.
- Slepushkin, V., et al., Large-scale purification of a lentiviral vector by size exclusion chromatography or Mustang Q ion exchange capsule. Bioproc J, 2003. 2(5): p. 89-95.
- Hernandez, R., Continuous manufacturing: A changing processing paradigm, in BioPharm International. 2015.
- Jacquemart, R., et al., A Single-use Strategy to Enable Manufacturing of Affordable Biologics. Comput Struct Biotechnol J, 2016. 14: p. 309-18.